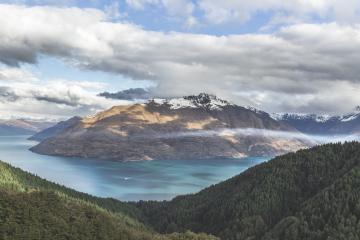
Supporting the Implementation of NGSS through Research: Pre-Service Teacher Education
Mark Windschitl, University of Washington
Christina Schwarz, Michigan State University
Cynthia Passmore, University of California, Davis
The Next Generation Science Standards (NGSS) and its parent document the Framework for K-12 Science Education (National Research Council, 2012) are the products of decades of research on how students learn science, and reflect the growing scholarship on the knowledge-building practices that characterize the discipline. The NGSS is presented to the science education community as a carefully designed yet imperfect text, as a promising lever for change but insufficient to that task without other parts of the system accommodating the effort. Pre-service preparation is a key part of that system.
But the context for preparation that exists today is itself in need of reform. Currently, “instruction about instruction” in many pre-service preparation programs is largely under-informed by the knowledge bases on teacher or student learning (Rand, 2002; US Department of Education, 2008). The quality of teacher education in science varies widely from one program to another, and in many programs there are few opportunities for learning about evidence-based teaching methods (Adams & Krockover, 1997; Clift & Brady, 2005). Novices’ exposure to pedagogy is often limited to the past experiences, skills, and worldviews of their instructors and cooperating teachers (Ball, Sleep, Boerst, & Bass, 2009; Deussen, Coskie, Robinson, & Autio, 2007; Little, 1990), and what is learned is haphazard. These conditions, in many ways, are not the products of choices made by individuals in programs, but rather they emerge from a larger system of constraints that are inherent to the culture of teacher education and to gaps in the field of science education research. For instance, problematic issues include the lack of opportunities for teacher educators to learn from one another, the absence of a shared “core set” of PK-12 instructional practices, and the limited use of effective teacher education pedagogies (e.g., deconstructing and modeling instructional practices, allowing opportunities for rehearsals of practice by novices, creating beginners’ tools for the design of instruction, providing principled feedback on attempts at practice).
We suggest that the Next Generation Science Standards be considered an opportunity for the professional development of pre-service educators. We describe below how this document might be used to expand the pedagogical repertoire of those who teach teachers of science. First, however, we describe the limits of the NGSS in terms of guiding instruction.
The Next Generation Science Standards Do Not Tell Us How to Teach
The NGSS tells us what kinds of ideas should take center stage in classrooms and they feature science practices as the active context for student learning. But the NGSS provide little insight into how a teacher might design and enact learning experiences for students. We must rely on other literatures that fill in the gaps to inform teaching methods, primarily about how to support all students’ learning of science. There are principles of powerful teaching for which strong consensus exists among researchers and knowledgeable practitioners. Among these are:
- organizing instruction around intellectually substantive and complex phenomena rather than taking a “basics first” approach (see for example Lehrer & Schauble, 2005; Palincsar & Magnusson, 2001),
- eliciting students’ ideas on a regular basis to shape instruction (see for example, Hammer, Goldberg, & Fargason, 2012; Minstrell & Kraus, 2005).
- making students’ thinking visible so that their ideas/reasoning/experiences become resources for others in the class (see for example, Michaels, Sohmer, O’Connor, & Resnick, 2009; Radinsky, Oliva, & Alomar, 2010),
- providing tools that allow students to revise their thinking over time (see for example, Passmore, & Stewart, 2002; Schwarz et al., 2011).
- scaffolding talk, reading and writing — in particular students’ attempts at evidence-based explanations (see for example, McNeill, 2009; Smith, Maclin, Houghton, & Hennessey, 2000),
- making explicit the “rules of the game” with regard to academic discourse and its relation to everyday language (see for example, Mercer, Dawes, Wegerif, & Sams, 2004; Nasir, Rosebery, Warren, & Lee, 2006), and
- fostering meta-cognition as a habit of mind (see for example, Bransford, Brown, & Cocking, 2004; Brown & Campione, 1994).
Our point here is that the standards are only one resource for preparing educators about instruction. And as history shows us time and time again, depending upon someone else to develop curriculum that supports rigorous, responsive, and equitable teaching is naïve. This is the work that is now before us as a field and a large part of that work will be to prepare pre-service teachers for the challenges ahead.
Implications for Pre-Service Instruction
Studying the framework. The NRC’s Framework document (2012) is a learning opportunity for pre-service teacher educators. This document lays out the rationale for why particular science ideas, scientific practices, and cross-cutting concepts were included in the NGSS. Even more importantly it describes research about students’ science learning that can inform a teacher educator’s theories of action about instruction. If pre-service educators could study the basis for the NGSS, it would help them refine their own pedagogy and become more flexible in how they adapt instruction to different grade levels or science domains. They should read the Framework with colleagues who share responsibility for science preparation and develop a set of conceptual frames or tools that can guide some of their programmatic work and teaching. The Framework contains references to many of the original research texts that explain, at length, ideas about instruction, learning, or science itself.
Constructing a sharable portfolio of ambitious practice. Just as there are scientific practices and teaching practices, there are also practices that are characteristic of teacher educators. These include modeling strong examples of science teaching to novices, showing how to attend to the thinking of students, and providing feedback to novices as they attempt to approximate instruction. The work of teacher educators includes instruction about curriculum materials and their design and about assessments and their relationship to teaching and learning. All of this must now be considered within the context of the NGSS. How would teacher educators develop these new understandings of practice? A compelling idea might be to expand one’s horizons in a public school classroom — for teacher educators themselves to enact a unit of instruction consistent with the NGSS, and doing so with young learners. We think of this, again, as an opportunity for professional development.
We imagine that a pre-service educator might collaborate with a local teacher to plan together a unit of instruction that is based on the NGSS. They then teach the unit together, documenting students’ learning across time, reflecting on lessons and revising their thinking about what to teach next. Artifacts from the unit could become part of a portfolio of learning. What would be included? Planning documents, lesson plans that are annotated with notes about how students responded to instruction, formative assessments, tools that were developed to support the science practices, and video of key lessons. The portfolio could be shared with critical friends, who offer feedback and encouragement. This whole process can be a rich learning experience, but it can also be humbling. The benefits, however, far outweigh the short-lived discomforts. We imagine how powerful it would be if a group of science teachers and teacher educators did this together, if they could learn from the experiences of their collective, develop a shared language about this type of teaching and even sets of tools to support novices in doing their own classroom work.
Sorting out how NGSS fits in with past reform efforts. Teacher educators can help novices understand the NGSS, but these novices will then be interning in schools where the faculty have likely wrestled with an assortment of previous reforms. Teacher educators will need to make clear connections between documents like Taking Science To School and the NGSS. For example, the four proficiencies listed in TSTS are entirely consistent with the goals of NGSS. On the other hand, the de-emphasis of the idea of “inquiry” and the new focus on scientific practices (from the Framework) can be seen as a conflict with what was championed in earlier reform documents (i.e. the previous Standards, the Benchmarks for Science Literacy, etc.). We recommend that the science education community create straightforward statements about how the NGSS fits with current and past reforms, and that this document be made available to teachers and teacher educators. If cooperating teachers do not see the links and distinctions across these documents, it will be hard for them to mentor their interns using the NGSS as one guide for the design of instruction.
The suggestions above signal that science teacher educators must engage with the NGSS in substantive ways that go well beyond familiarizing themselves. They would benefit greatly from professional development that is contextualized in settings similar to those their pre-service students will encounter.
Unresolved Issues
We see two issues, invoking both opportunity and concern that will become more prominent with the adoption of the NGSS. The first of these deals with accountability. Part of pre-service apprenticeship includes being evaluated by others, both on the basis of students’ test performances and on classroom observations. These evaluations can influence their employment prospects and their professional status. But we do not yet know if or how these evaluation measures used by principals or teacher education supervisors are going to be “tuned,” at least in part, to the goals of the NGSS. We don’t know if the widely used Teacher Performance Assessment will be made consistent with the NGSS. And, we do not yet know how state level science tests will change and what it will imply for learning to teach. There is plenty of uncertainty to go around, and much of it is because the NGSS do not tell us how to teach, but rather what is important to teach. Until clear images of ambitious and equitable teaching come into focus for the science education community, we will continue to struggle with how we evaluate novices, provide feedback, and as a field, engage in the continual improvement of teaching.
The second issue is as problematic in scale and consequence as the first. At the elementary level, science in many districts is not even part of the curriculum. Some students are not exposed to science until the fourth grade or even middle school. We are seeing first hand in our own research enormous inequities emerging between those who have access to rich science experiences early in school and those who don’t. This is essentially programming our students for failure. The present moment seems to be our best chance to raise the profile of science at the elementary level, perhaps by linking it with literacy and mathematics. Teacher educators at the elementary level could take the lead in articulating what the NGSS looks like in PK-6 classrooms. Case studies might include integrating the full range of Common Core areas — science, reading, writing, speaking, mathematics. Such a goal brings us back again to the challenge of inferring the kinds of teaching required to realize the goals embodied in the NGSS.
Summary
The NGSS provides opportunities to re-consider how we educate teachers. Every stakeholder is a learner in this picture, but none of what we recommend here can happen through the efforts of individuals working alone. This is not about tweaking the science education system; the preparation of excellent educators is a systems-level phenomenon. This means that entire institutions and the actors, resources, norms and practices that make up these systems must coordinate this effort to change. Sharing a clear vision of rigorous and equitable teaching would be a strong first step.
References
Adams, P. E., & Krockover, G. H. (1997). Beginning science teacher cognition and its origins in the preservice secondary science teacher program. Journal of Research in Science Teaching, 34, 633–653.
Ball, D. L., Sleep, L., Boerst, T., & Bass, H. (2009). Combining the development of practice and the practice of development in teacher education. Elementary School Journal, 109(5), 458 – 474.
Bransford, J. D., Brown, A. L., & Cocking, R. R. (2000). How people learn: Brain, mind, experience, and school (expanded edition). Washington, DC: National Academies Press.
Brown, A.L., & Campione, J.C. (1994). Guided discovery in a community of learners. In K. McGilly (Ed.), Classroom lessons: Integrating cognitive theory and classroom practice (pp. 229-270). Cambridge, MA: MIT Press/Bradford Books.
Clift, R. T., & Brady, P. (2005). Research on methods courses and field experiences. In M. Cochran-Smith & K. Zeichner (Eds.), Studying teacher education (pp. 309–424). Mahwah, NJ: Erlbaum.
Deussen, T., Coskie, T., Robinson, L., & Autio, E. (2007). “Coach” can mean many things: Five categories of literacy coaches in Reading First (Issues & Answers Report). Retrieved from http://ies.ed.gov/ncee/edlabs.
Hammer, D., Goldberg, F., & Fargason, S. (2012). Responsive teaching and the beginnings of energy in a third grade classroom. Review of Science, Mathematics and ICT Education, 6(1), 51-72.
Lehrer, R., & Schauble, L. (2005). Developing modeling and argument in elementary grades. In T. A. Romberg, T.P. Carpenter, & F. Dremock (Eds.) Understanding mathematics and science matters (pp. 29-53). Mahwah, NJ: Lawrence Erlbaum Associates.
Little, J.W. (1990). The persistence of privacy: Autonomy and initiative in teachers’ professional relations. Teachers College Record, 91(4), 509-536.
McNeill, K. L. (2009). Teachers’ use of curriculum to support students in writing scientific arguments to explain phenomena. Science Education, 93(2), 233-268.
Mercer, N., Dawes, L., Wegerif, R.,& Sams, C. (2004). Reasoning as a scientist: ways of helping children to use language to learn science. British Education Research Journal, 30, 359- 377.
Michaels, S., Sohmer, R., O’Connor, M.C., & Resnick, L. (2009). Guided construction of knowledge in the classroom: The troika of talk, tasks, and tools. In, Schwartz, B. (Ed.), Advances in Learning and Instruction (pp. 105-129). Paris: Elsevier Press.
Minstrell, M. & Kraus, P. (2005). Guided inquiry in science classrooms. In M.S. Donovan & J. Bransford (Eds.), How students learn science in the classroom (pp. 475-514). Washington DC: National Academies Press.
Nasir, N. S., Rosebery, A. S., Warren, B., & Lee, C. D. (2006). Learning as a cultural process: Achieving equity through diversity. In R. K. Sawyer (Ed.), The Cambridge handbook of the learning sciences (pp. 489–504). New York, NY: Cambridge University Press.
Radinsky, J., Oliva S., & Alamar, K. (2010) Camila, the earth, and the sun: Constructing an idea as shared intellectual property. Journal of Research in Science Teaching, 47(6), 619–642.
Schwarz, C., Reiser, B., Acher, A., Kenyon, L.,& Fortus, D. (2011). MoDeLS: Challenges in defining a learning progression for scientific modeling. In A. Alonzo& A. Gotwals (Eds.) Learning progressions in science, current challenges and future directions (pp. 101-138). Sense Publishers: Rotterdam.
Smith, C. L., Maclin, D., Houghton, C., & Hennessey, M. G. (2000). Sixth-grade students’ epistemologies of science: The impact of school science experiences on epistemological development. Cognition and Instruction, 18(3), 349–422.
National Research Council (2012). A framework for K-12 science education: Practices, crosscutting concepts, and core ideas. Washington, DC: The National Academies Press.
Palincsar, A. S. & Magnusson, S. J. (2001). The interplay of first-hand and text-based investigations to model and support the development of scientific knowledge and reasoning. In S. Carver & D. Klahr (Eds.), Cognition and instruction: Twenty five years of progress (pp. 151-194). Mahwah, NJ: Lawrence Erlbaum.
Passmore, C., & Stewart, J. (2002). A modeling approach to teaching evolutionary biology in high schools. Journal of Research in Science Teaching, 39(3), 185 – 204.
Rand (2002). Reading for understanding: Toward an R&D program in reading comprehension. Santa Monica, CA: Author.
Rosebery, A., Ogonowski, M., DiSchino, M., & Warren, B. (2010). “The coat traps all your body heat”: Heterogeneity as fundamental to learning. Journal of the Learning Sciences, 19(3), 322-357.
U.S. Department of Education (2008). The final report of the National Mathematics Advisory Panel. Washington, DC: Author.
Suggested Citation
Windschitl, M., Schwarz, C., & Passmore, C. (2014). Supporting the implementation of the Next Generation Science Standards (NGSS) through research: Pre-service teacher education. Retrieved from https://www.narst.org/blog/ngss-preservice